Earth formed from the Sun’s protoplanetary disk about 4.6 billion years ago. In the beginning, it was a molten spheroid with scorching temperatures. Over time, it cooled, and a solid crust formed. Eventually, the atmosphere cooled, and life became a possibility.
But how did all of that happen? The atmosphere was rich in carbon, and that carbon had to be removed before the temperature could drop and Earth could become habitable.
Where did all the carbon go?
The first 500 million years of Earth’s existence are informally called the Hadean eon. The name comes from Hades, the Greek God of the Underworld. Hades is also an informal name for Hell itself.
The Hadean eon is aptly named. Even after it began to cool and solidify, Earth was still scorching hot. The atmosphere contained 100,000 times the current level of atmospheric carbon. Early Earth was similar to Venus, where a thick atmosphere traps heat and keeps temperatures high. During the Hadean, Earth’s surface temperature would’ve exceeded 200 Celsius (400 F.)
Before Earth could cool, it had to scrub a lot of carbon from its atmosphere. But scientists have found it challenging to piece together events on the very young Earth. For one thing, the geological evidence is scant.
But a pair of researchers think they have a new explanation for removing all that atmospheric carbon, and it involves a type of rock that no longer exists.

A new research article titled “A wet heterogeneous mantle creates a habitable world in the Hadean” presents the team’s findings. The first author is Yoshinori Miyazaki, a Stanback Postdoctoral Fellow at Caltech. Jun Korenaga, a professor of Earth and planetary sciences at Yale, is the other author. The journal Nature published the study.
“This period is the most enigmatic time in Earth history,” said Korenaga in a press release. “We’re presenting the most complete theory, by far, for Earth’s first 500 million years.”
The Hadean was not only enigmatic but dynamic. The planet underwent a lot of changes during those 600 million years. But geological evidence shows that Earth’s surface environment was similar to present-day Earth’s by the middle of the Hadean. “Under what conditions a harsh surface environment could turn into a habitable one remains uncertain,” the authors write in their paper.
A few things had to happen before Earth could be habitable. Oceans had to form, plate tectonics had to start, and greenhouse gases had to be removed quickly from the atmosphere. “Somehow, a massive amount of atmospheric carbon had to be removed,” Miyazaki said. “Because there is no rock record preserved from the early Earth, we set out to build a theoretical model for the very early Earth from scratch.”
In Earth’s early days, it was a magma ocean, a sphere of molten rock and nothing else. During this phase, the planet was forming via accretion. In the accretion process, materials agglomerate together creating a large object over time. Earth took between 70 million and 100 million years to assemble. During that time, planetesimals slammed into the Earth-to-be, generating heat and keeping Earth in a molten state.
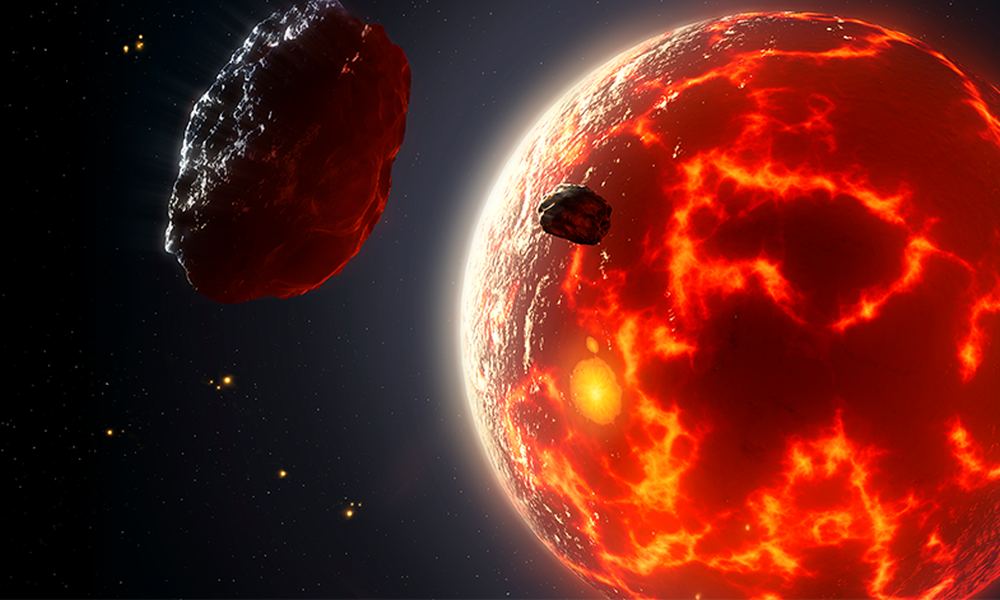
The magma ocean is an essential stage in the life of rocky planets. The liquid state allows heavier elements to sink to the core and lighter elements to “float” on top. This is how planets like Earth become differentiated into a core, a mantle, and a crust. The outer core is still molten to this day, and without it, Earth would have no protective magnetosphere and probably no life.
When Earth’s magma ocean solidified, it released massive quantities of greenhouse gases (GHGs) into the atmosphere. As a result, Earth’s early atmosphere contained a lot of CO2 and H2O. Those gases helped maintain the young planet’s extreme climate. For that climate to become more moderate within the timeframe of the Hadean, things had to change rapidly, in geological terms. Those greenhouses gases had to be removed, and the only repository for them was the rock. As part of Earth’s carbon cycle, carbon is sequestered into rock through transformation into carbonate minerals in ocean basins. From there, the carbonates become part of the mantle. (Some of the carbon we’re emitting now will be subducted and end up as diamonds in the distant future.)
But the question is about the time involved. If the climate was moderate and similar to modern Earth’s 4 billion years ago, the carbon sequestration had to be very efficient. How did it all work?
A kind of prehistoric rock played a role in making Earth habitable, according to Korenaga and Miyazaki. They’re called high-magnesium pyroxenites. The pair of researchers say none of these rocks exist on Earth today. But the rapid carbon removal model they developed—based on thermodynamics, fluid mechanics, and atmospheric physics—shows that these rocks must have existed. They even know what they would’ve looked like.
“These rocks would have been enriched in a mineral called pyroxene, and they likely had a dark greenish colour,” Miyazaki said. “More importantly, they were extremely enriched in magnesium, with a concentration level seldom observed in present-day rocks.”
Magnesium minerals have an affinity with carbon dioxide. They form carbonates which are then sequestered into Earth’s mantle. If there were enough high-magnesium pyroxenites, they could’ve helped account for the rapid removal of carbon from Earth’s atmosphere.
But that’s only part of the explanation for the the geologically rapid transformation of Earth’s atmosphere during the Hadean. Magnesium-rich minerals may have been plentiful, but something else needed to happen. Remember that the build-up of carbon in the atmosphere stemmed from the cooling of the magma ocean. As it cooled and solidified it released huge quantities of GHGs into the atmosphere.
During the Hadean eon, Earth had what’s called a wet mantle. The mantle is 3,000 km (1,900 mile) thick layer of rock. A wet mantle is one that contains a high proportion of water, and that water affects convection.
Earth’s mantle contains a lot of silicate minerals, and they were molten during the Hadean. Water lowers the melting point of silicates, keeping more of the silicates molten. Convection currents in the molten material meant that the mantle experienced convection. That means that the wet mantle experienced more convection, which brought more of the magnesium-rich minerals to the surface where it could react with carbon. In effect, the mantle surface recycled itself more rapidly, bringing new magnesium into contact with carbon more rapidly. Eventually that carbon was removed from the atmosphere and sequestered into the mantle.

For this to work, the mantle had to be chemically heterogenous. That means the composition wasn’t uniform, but instead consisted of different and diverse constituents. “Given that a habitable environment had emerged by 4.0?Ga, the Hadean evolution is explained more naturally by the mantle with a chemically
heterogeneous structure,” the authors write in their paper. “We propose that rapid recycling is possible with a chemically heterogeneous mantle…”
If the mantle had been more homogenous, the atmosphere might not have cooled so rapidly. That’s because a more homogenized mantle wouldn’t have developed the same type of lithospheric cap that promoted rapid plate movement. A homogenized mantle would’ve still developed a depleted lithospheric cap, but it would’ve been much thicker. The thicker cap would’ve slowed down plate movement, which would’ve slowed carbon sequestration. “Our results suggest that the chemically heterogeneous mantle is more compatible with creating a habitable environment by the end of the Hadean,” the authors write.

A third thing had to happen for Earth to be habitable. Habitability required not only active plate tectonics and removal of GHGs from the atmosphere, bit also required oceans.
The model the authors developed also accounts for ocean formation. A heterogeneous mantle has more convection, which exposes more magnesium-rich rocks to the atmosphere. That’s what removes GHGs from the atmosphere, cooling to to more modern day temperatures. But the more rapid convection in the mantle also releases more volatiles into the atmosphere. The amount of volatiles released “… exceeds a threshold of 0.05?ocean mass to form water oceans when the atmosphere contains 200?bar of CO2, so the presence of ocean is plausible immediately after the solidification of a magma ocean,” they explain.
Were the higher temperatures a problem? The temperature was high enough to vaporize the water, but the pressure was high, too. “The surface temperature exceeds 100?°C owing to the greenhouse effect, yet liquid water is stabilized by high atmospheric pressure,” the authors explain.
There’s another fascinating result of this work: the model not only shows how the atmosphere cooled and became habitable more quickly, it also shows how the “weird” magnesium-rich rocks created more chemicals necessary for biology earlier in Earth’s history.
“As an added bonus, these ‘weird’ rocks on the early Earth would readily react with seawater to generate a large flux of hydrogen, which is widely believed to be essential for the creation of biomolecules,” Korenaga said.
“A high iron-rich olivine content in crust of the chemically heterogeneous mantle would promote serpentinization reactions, which has an important implication for the earliest life on Earth,” the researchers say. Serpentinization is when seawater comes into contact with ultramafic rock. Serpentinization produces hydrogen, which then reduces atmospheric CO2 to methane. Early life forms relied on methane. “Serpentinization releases hydrogen and methane by reducing water, and the anaerobic oxidation of methane is considered to have supported nascent life forms before the beginning of photosynthesis,” the paper states.

The serpentinization and production of biomolecules in the ancient mante’s crust would be similar to a rare type of modern, deep-sea thermal vent, called the Lost City hydrothermal field, located in the Atlantic Ocean. Scientists are very interested in the Lost City hydrothermal field because of its abiotic production of hydrogen and methane. Scientists think the serpentinization that takes place there is similar to the early Earth. Like the early Earth, the Lost City also produces hydrogen and methane fundamental to microbial life. Some scientists think that life on Earth may have originated in ancient vents like the ones at the Lost City.
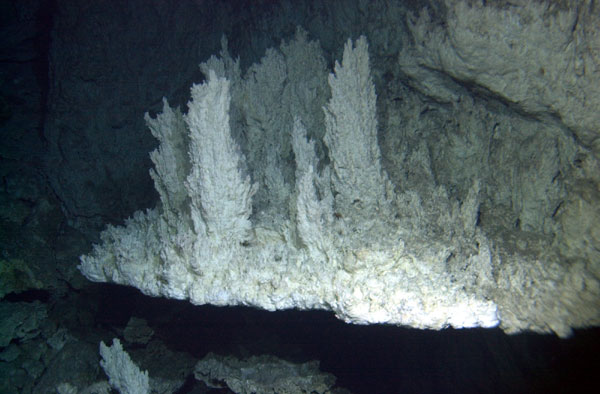
“Our theory has the potential to address not just how Earth became habitable, but also why life emerged on it,” Korenaga said in the press release announcing their work.